SI engine Idle Speed Control
The aim of idle speed control (ISC) is to keep the engine speed during idling close to its reference value, regardless of changes of engine load due to the engagement of various accessories such as air conditioning, alternator, and power steering. More effective load disturbance rejection provides the application of lower reference idle speed without the risk of engine stalling, and thus leads to lower fuel consumption and reduced emissions. A proportional-integral (PI) controller is commonly used in automotive practice to control the engine idle speed through the throttle angle input. The PI controller action may effectively be augmented by a feedforward action of a load torque compensator, where the load torque can be reconstructed directly from the engine accessory devices measurement signals, such as the alternator current and air conditioning unit status. However, the accessory sensors are often not installed in production vehicles or their readings may not be sufficiently accurate or may not be available to the Engine Control Unit (ECU). Therefore, instead of utilizing the PI controller augmented with the feedforward compensator, higher-order controllers such as PID or polynomial controllers may be used in order to control a greater number of relevant engine dynamics modes. Generally, the main limitations of higher-order controllers include sensitivity to noise, and to engine modeling errors.
In order to provide a favorable tradeoff between the load torque disturbance rejection performance and noise sensitivity, an adaptive idle speed controller has been designed. It relies on the constant-gain PI speed controller extended with an adaptive Kalman filter for load torque estimation and compensation, as shown in Fig. 1. The adaptation mechanism is based on a load torque change detection algorithm. The overall adaptive controller is modular and can, thus, be tuned and verified in a straightforward way. The proposed adaptive idle speed controller has been extensively verified against other conventional controller structures. The verification has been carried out on the experimental setup of a 14 HP V2 SI engine.
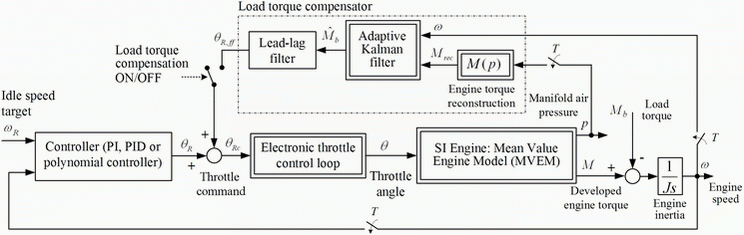
Fig. 2 shows the comparative responses of ISC systems with the proposed adaptive controller and the PI, PID and polynomial controller for the case of load torque step change of 4 Nm (15% of the rated engine torque). For the particular case of small V2 engine, the idle speed target is set to 1200 rpm in order to facilitate smooth engine operation. The load engagement responses in Fig. 2 show that the ISC system based on the PI controller alone is characterized by a well-damped, but rather slow load torque step recovery with a large engine speed drop of 320 rpm. By applying the more complex linear controllers such as the PID or polynomial controller, the engine speed drop magnitude and duration is significantly reduced (to approximately 200 rpm), but the noise levels in throttle command are notably increased (especially in the case of PID controller), and the response may exhibit an overshoot (when polynomial controller is used). The controller with adaptive load torque compensator effectively reduces the engine speed drop due to accurate estimation of abrupt load torque change and corresponding fast throttle command reaction. Since the Kalman filter adaptation is impulsive (Kalman filter gains are quickly returned to low steady-state values after the change detection), the estimated load torque and throttle command are characterized by notably improved signal-to-noise ratio compared to the PID controller, and the response overshoot (and robustness) issue associated with polynomial controller utilization is effectively overcome.
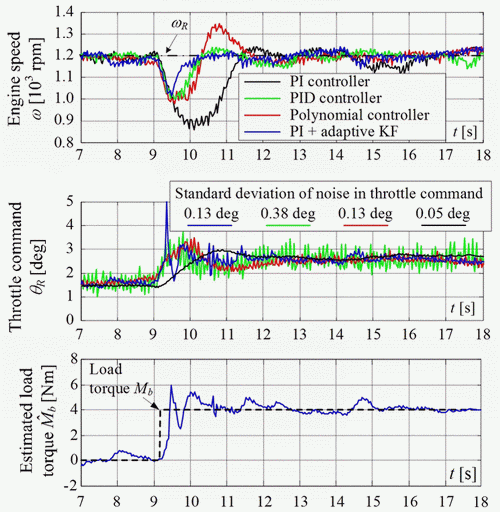
The proposed adaptive load torque compensator has also been compared with a feedforward load torque compensator (FFC), which is based on the "measurement" of engine load-related variables (e.g. alternator current measurement). The results of comparative experimental analysis of the adaptive Kalman filter-based load torque compensator and the feedforward load torque compensator without and with load torque filtering/reconstruction delay are shown in Fig. 3. As can be expected, the utilization of "idealized" load torque compensator without reconstruction delay provides the best performance. However, it is important to note that the commanded signal has a very similar trace for the systems with feedforward compensator and adaptive Kalman filter-based compensator, which is characterized by an abrupt, high-magnitude controller action after the load torque change. The main difference is that the adaptive Kalman filter-based compensator reacts with an inevitable delay (needed to provide robust load torque change detection). When the adaptive compensator is verified against the FFC comprising the filtering/reconstruction delay, their control performances become comparable. This suggests that the proposed adaptive compensator provides a kind of ultimate performance for the case when the load torque cannot be reconstructed (but rather estimated).
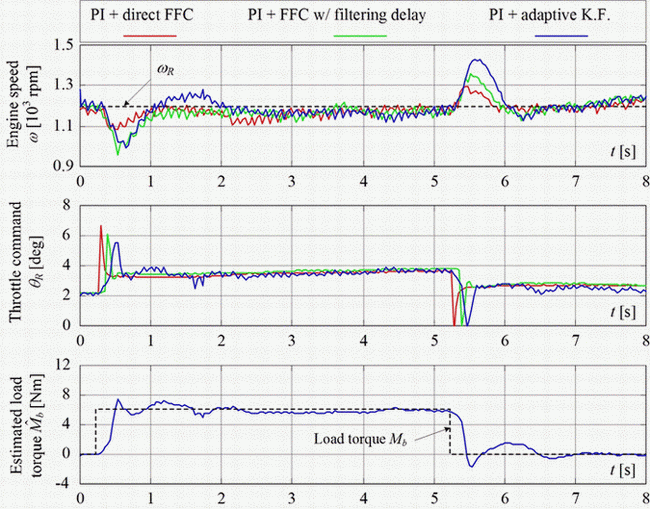
Publications
Lambert Academic Publishing, Saarbrücken, 2011. Adaptive Kalman Filter-Based Load Torque Compensator for Improved SI Engine Idle Speed Control
IEEE Transactions on Control System Technology, Vol. 17, No. 1, pp. 98-110, 2009. State Estimation of Automotive Drive with Control Applications
Doctoral thesis (in Croatian), Faculty of Mechanical Engineering and Naval Architecture, University of Zagreb, Croatia, 2007. Identification and Speed Control of SI Engine for Idle Operating Mode
SAE paper #2004-01-0898, 2004 SAE World Congress, Detroit, MI, 2004.